Rising power demand and the integration of renewables are causing increasing congestion on AC transmission lines. Physically expanding AC capacity is not easy or cheap. By converting existing AC lines to DC, however, power levels can be boosted significantly without adding new lines.
Peter Lundberg, Bjorn Jacobson ABB Power Grids, Grid Integration, Ludvika, Sweden, peter.lundberg@se.abb.com, bjorn.jacobson@se.abb.com; Vinothkumar K, Gaurav-Kumar Kasal ABB GISPL, Chennai, India, vinothkumar.k@in.abb.com, gaurav-kumar.kasal@in.abb.com; Shanthakumar MS ABB India Ltd., Gurgaon, India, shanthakumar.MS@in.abb.com; Abhay Kumar ABB AB, Ludvika, Sweden, abhay.kumar@se.abb.com
All around the world, and especially in developing countries, the demand for electrical power is rising. Along with climate change concerns, this increasing demand is fueling a rapid expansion in generation from renewable energy sources. In fact, wind and solar are the fastest-growing electrical energy sources in the world today. However, the additional electrical energy associated with these trends has to be transported to the consumer, which means existing AC transmission networks are now struggling to cope with power transfer levels above those for which they were designed. To complicate matters, renewable energy sources are intermittent, which makes economic planning of transmission capacity difficult.
One obvious solution to transmission congestion is to construct additional AC lines. However, quite apart from the capital costs, new rights of way may require environmental impact and engineering assessments, and a long list of licenses, agreements, authorizations and compulsory land purchases. Therefore, transmission system operators (TSOs) look for alternative technologies that can enhance power transmission and integrate renewables while maximizing the use of existing rights of way.
An alternative to AC
One alternative to AC is high-voltage direct current (HVDC) transmission. HVDC has many advantages over AC, such as enhanced power transmission capacity up to the thermal limit [1], low losses, ease of flexibility and controllability (both of which help counteract any disruption arising from the connection of renewables), less demanding right of way requirements, etc. In particular, HVDC technology based on voltage sourced converters (VSCs) is capable of independent control of real and reactive power, voltage support to the connected AC system and black-start capability. To understand the challenges and opportunities involved in the conversion of an existing AC transmission infrastructure into HVDC, some case studies will be examined and their major technical aspects discussed.
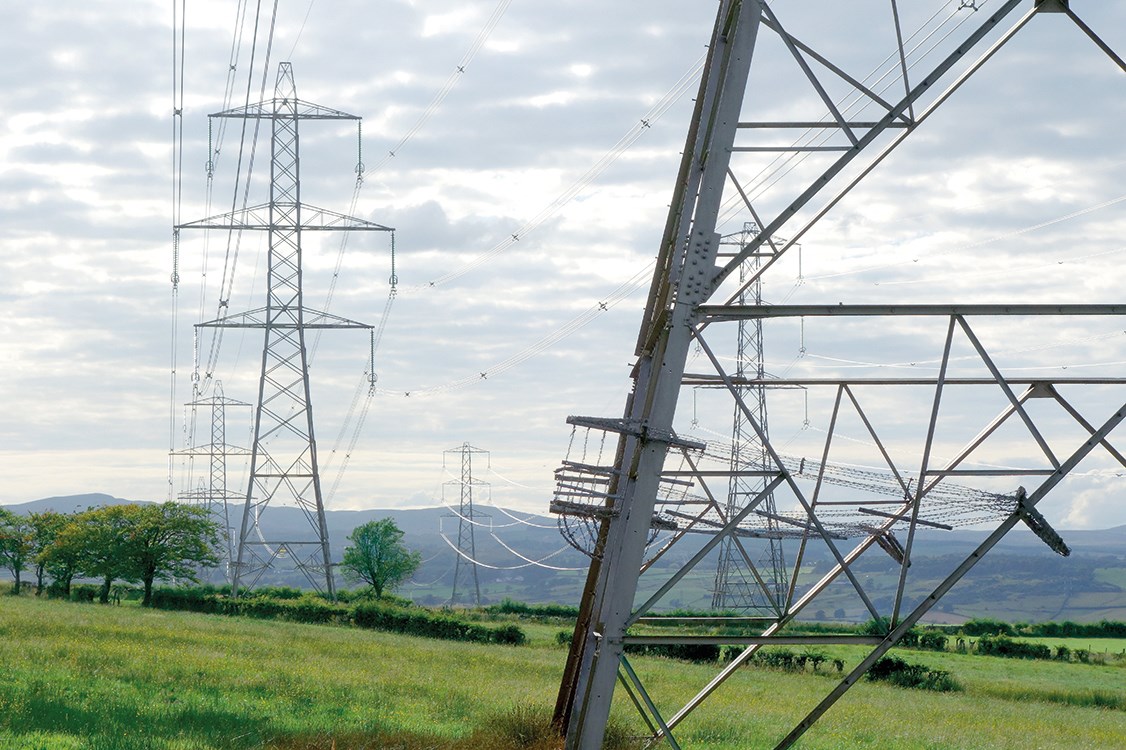
Factors that dominate AC to HVDC conversion
Before converting an AC line to HVDC, careful consideration must be given to a range of environmental, engineering and economic factors→2.

When a decision has been made to proceed with the conversion, the HVDC configuration must be decided: symmetric/asymmetric monopole or bipole or a hybrid that suits the tower configuration and the clearances available→3. For example, a horizontal single-circuit AC transmission line can be replaced with either one or two symmetrical monopole HVDC lines. A bipole configuration is also an option. In the case of a double circuit or multi-circuit, either one or several AC systems can be converted to HVDC as shown in→3. However, replacement is not straightforward and modifications may have to be made to the tower structure, insulators and conductors [1]. The AC ceramic insulators are generally replaced with high resistivity toughened glass (HRTG) or composite insulators to meet the clearance requirements [2].
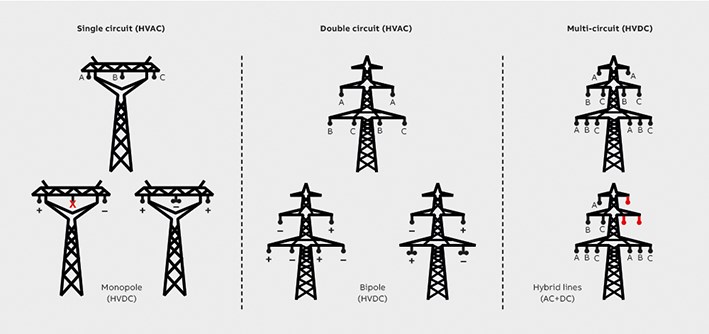
Existing conversions
AC to HVDC conversions to enhance the power transmission capacity of existing rights of way are ongoing. The first known example is the UltraNet project in Germany where a 400 kV AC line will be converted. Other lines have also been studied with a view to conversion.
In the UltraNet project, two 380 kV AC and two 110 kV AC systems run between North Rhine-Westphalia and Baden-Württemberg over a distance of 340 km [3]. One of the 380 kV AC systems has been converted to a 380 kV DC system, which will facilitate a power transmission capacity of approximately 2,000 MW from wind farms in the North Sea to the industrial towns in the south of Germany→4.
![04 AC to DC conversion in the UltraNet project in Germany [3]. © Amprion TransnetBW.](https://resources.news.e.abb.com/images/2018/12/6/0/m8036-04-EN.jpg)
The conversion has significanty increased power capacity. Raising the DC voltage can increase capacity but care must be taken as ambient temperatures cause retained conductors to sag, endangering clearances – for example, an extra sag of around 1.3 m will occur in a conductor at 40 °C compared with its catenary at 0 °C [2]→5.
![05 Power transmission capacity of existing right of way based on DC voltage selection and ambient temperature [2].](https://resources.news.e.abb.com/images/2018/12/6/0/m8036-05-EN.jpg)
A further conversion example is found in the Angle-DC project [4]. Here, a 33 kV AC connection between North Wales and Anglesey in the UK has been converted to a 27 kV symmetric monopole HVDC line to achieve a 23 percent increase in power transmission capacity for an operating temperature of 50 °C. By allowing a cable operating temperature of 65 °C, capacity can be increased by 35 percent.
Selection of DC voltage
The string length determines the available clearance, which governs the maximum DC voltage that can be carried. The thermal rating of the conductor determines the current-carrying capacity of the transmission system. The DC voltage selected and the thermal current rating of the conductor together determine the maximum power transmission capacity of the line after conversion.
The length of the insulator string depends on the creepage distance requirement. Creepage distances vary depending on local conditions, such as salt deposition from sea spray or atmospheric industrial pollution load. The creepage distance requirement for HVDC is higher than that of AC.
For conversion of a typical 220 kV AC transmission system – assuming that the string length of 2,030 mm is retained to maintain allowable sag during operation – the DC voltage will be in the range of 146 to 206 kV for a symmetrical monopole HVDC configuration. The particular value will depend on the DC creepage requirement for the pollution zone in which the line finds itself. This is depicted in→6 for two different HRTG insulator arrangements from Sediver – C170DR and C195DR.

[4] “Angle-DC: 2015 Electricity Network Innovation Competition.” Available: https://www.ofgem.gov.uk/sites/default/files/docs/angle_submission.pdf
[5] M.I.Khan, R.C.Agrawal, “Conversion of AC line into HVDC,” IEEE PES Conference and Exposition, Durban, South Africa, July 2005.
Accordingly, the power transmission capacity ranges between 520 MW and 740 MW when the thermal current rating of the conductor is assumed to be 1,800 A with a symmetrical monopole HVDC configuration→7.
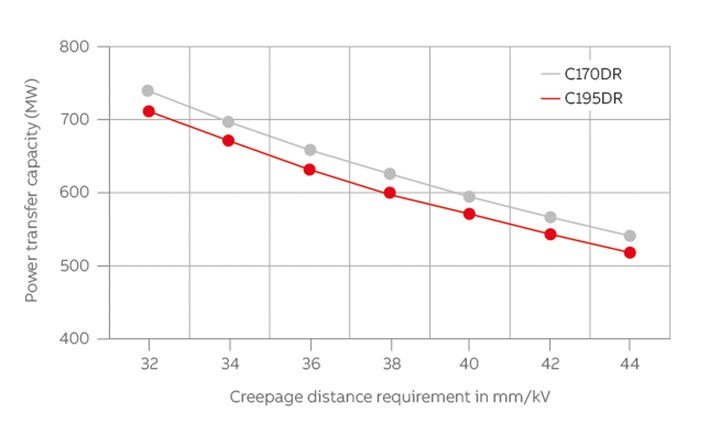
The use of composite insulators can further improve the power transmission capacity as they are less affected by pollution and they also allow a higher DC voltage to be used. Suitable modification to the cross-arms arrangements can also improve clearance and allow higher DC voltages→8. The thermal current rating, and thus power transmission capacity, of the conductor can be increased either by adding more sub-conductors or replacing it with a new conductor.
![08 Conversion to higher DC voltage by suitable modification of the tower structure [5].](https://resources.news.e.abb.com/images/2018/12/6/0/m8036-08.jpg)
AC to DC in the future
Given that certain basic economic, engineering and environmental conditions can be fulfilled, the conversion of an existing AC transmission line to HVDC can significantly increase the power capacity of an existing right of way. Higher DC voltages deliver higher capacities but account must be taken of conductor sagging and the effect of pollution levels on the creepage distance, which is one of the main determinants of the maximum DC voltage allowed. Tower modification or the use of composite insulators may enable an even higher DC voltage to be chosen. Conductor thermal ratings can be increased by adding more sub-conductors or by replacing the conductor, resulting in higher power transmission capacity.
As demand for capacity on transmission lines continues to increase, it is expected that more AC lines will be converted to HVDC to take advantage of the higher powers that can then be carried without having to build a new line.
References
[1] M. Hausler et al., “Converting AC power lines to DC for higher transmission ratings,” ABB Review, 3/1997, pp. 4-11.
[2] J. Lundkvist et al., “Feasibility study for converting 380 kV AC lines to hybrid AC/DC lines,” EPRI High-Voltage Direct Current & Flexible AC Transmission Systems Conference, Westminster, USA, Nov. 2009, pp. 1-25.
[3] “Project description: Ultranet.” Available: https://www.amprion.net/Grid-expansion/Our-Projects/Ultranet/
[4] J. Lundkvist et al., “Feasibility study for converting 380 kV AC lines to hybrid AC/DC lines.” Available: https:// library.e.abb.com/ public/72440057976 5e22cc12577200041 00e5/Convertion%20of%20AC%20lines%20to% 20hybrid%20AC_ DC%20lines_09MP0519.pdf
[5] “Angle-DC: 2015 Electricity Network Innovation Competition.” Available: https://www.ofgem.gov.uk/sites/default/files/docs/angle_submission.pdf
[6] M.I.Khan, R.C.Agrawal, “Conversion of AC line into HVDC,” IEEE PES Conference and Exposition, Durban, South Africa, July 2005.